Mechanobiological challenges related to hydrogel-based bioprinting technology for manufacturing novel 3D cell culture models
Department of Dental Materials and Biomaterials Research (ZWBF), Uniklinik RWTH Aachen
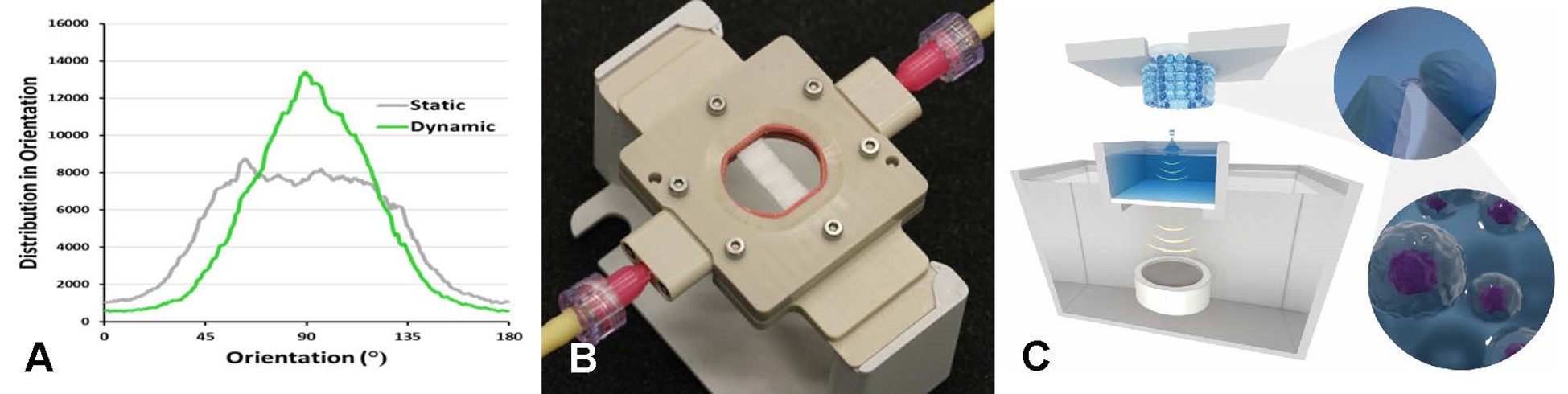
Project overview. (A) Collagen fibers align in response to defined dynamic stress application. (B) shows a bioreactor to investigate the effect of fluid shear stress on epithelial cells. (C) The acoustic bioprinting principle is used for realization of advanced 3D in vitro epithelial models. Single cells and cell clusters can be precisely printed in 3D and are subjected to much less shear stress during printing due to the nozzle-less technology developed in our lab.